The volume of an ‘ice cream cone’ is that of the hemisphere, 2/3 πr3, plus that of the cone, πr2h/3. If the angle at the bottom of the cone is 30°, then we can make a right triangle with angle 15°. It’s height is r/tan(15°), or 3.372r. This makes the volume of the ice cream cone 5.372rπr3/3. This is 500ml, or 500cm3. We can then make the equation 5.372rπr2/3=500cm3, which solves to r=9.428. Now, the surface area of the hemisphere is 3πr2, and that of the cone, πr(r+√(h2+r2)). But we must subtract 2πr2 for the 2 circles that are not exposed. That leaves us with 2πr2 + πr√(h2+r2). Plug in r=9.428 and h=3.372r to get our answer. 1601cm2.
V=500cm^3=1.791\pi r^2\\[8pt] r=9.428cm\\[16pt] SA=2\pi r^2+\pi r\sqrt{h^2+r^2}\\[8pt] SA=2\pi r^2+\pi r\sqrt{1.07177r^2}\\[8pt] SA=5.732\pi r^2\\[8pt] SA=1601cm^2Tag: solution
Solution to Fascinating Frequencies 6
We’re trying to figure out the minimum amount of fuel that needs to be burned in order to bring a rocket back into geostationary orbit. Firstly, how much energy is released by the explosion? Sodium is an Alkali with atomic weight 23, and Fluorine is a Halogen with atomic weight 19. This means they are in the perfect proportions to explode. There is no precise amount of energy this explosion will produce. However, we’re trying to find how much fuel is necessary to ensure the scientists can get back to orbit. So we take the maximum. The first ionization energy of sodium is 4.26 eV. The electronegativity of fluorine is 3.98 eV. 4.26-3.98=0.28 electron volts of energy released per reaction. There are 6.022×1025 of each. The total energy released is 2.702×106 m2kg/s2 of energy. This produces a force of 2.702×103 metres kilograms per second. Divide this by the mass of the station, 500Mg, and you get a total acceleration of 5.404 ×10-3 metres per second of delta v. The most fuel efficient way to undo this is to simply wait until the rocket comes back and then switch back. This is because the rocket’s new orbit will still include the spot it changed orbit’s at, and in the case of prograde burns, this will be it’s perigee. The perigee also happens to be the time when retrograde burns are most efficient. The release of 5.404 ×10-3 metres per second of delta v requires a push of, guess, 2.702×103 metres kilograms per second of force, same as last time. This requires, again, 2.702×106 m2kg/s2 of energy. The liquid fuel used by the scientists, oxygen and hydrogen, releases 1.418 m2/s2. This does not seem to be the correct unit, it is missing the mass. That is because the mass is the unknown. We divide the total energy by this to find the total mass, 1.906×106 kilograms. But this weighs far more than the station! The scientists do not have enough fuel to get back into orbit.
Solution to Sophisticated Streetcar
We’re trying to figure out when you should jump off of a streetcar to arrive as soon as possible. The distance you need to run is equal to the square root of the sum of the square of the distance of your destination to the streetcar track and that of the streetcar track before the shortest run. The former is simply 1 million square metres. Let the second be x squared. The distance is then defined by √(1,000,000+x2). The point to jump off is thus when the derivative of this is equal to 5/15, or 1/3. This relationship is shown by the equations below and is true when x is 250√2. The equation gives 750√2 at that point, so divide that by 5 m/s to see how long you run for. Our answer is 150√2, or 212.1, seconds of running.
\frac d{dx}\sqrt{x^2+1\,000\,000}=\frac13\\[8pt] \frac d{dx}\sqrt{x^2+1\,000\,000}=\frac x{\sqrt{x^2+1\,000\,000}}\\[8pt] \frac x{\sqrt{x^2+1\,000\,000}}=\frac13\\[8pt] x=250\sqrt2Solution to Fascinating Frequencies 5
We’re trying to find the frequency at which a gear on a fidget toy is spinning. Firstly, the centres of each gear form a hexagon shape. Since each gear has radius 10 centimetres, this hexagon has side length 20 cm. In a hexagon, the distance between two opposite vertices is double that of the side length. So the outermost gear is 40 cm from the innermost. Centripetal force is π2rλ/s inwards. r is the distance between the point that’s moving and the point it’s moving around. λ is the frequency at which it is moving around. We can move around the elements around to see that λ = Fs/π2r, where F is centripetal force. Plug in the knowns to see that the gear is moving around 0.2533 times per second. If you tried making the device, you’d see that only three gears spin. That’s because, for the anchored gear to stay in position, the motion of the hexagon has to exactly balance it’s rotation. That means 2 other gears will be balanced, but the other 3 will move at double speed. Half from the rotation of the hexagon and half from their own rotation. So the rotation from the hexagon, 0.2533 rotations equals that at which the gear is spinning. Therefore the gear is moving at 0.2533 rotations per second.
Solution to Gangbuster Geometry
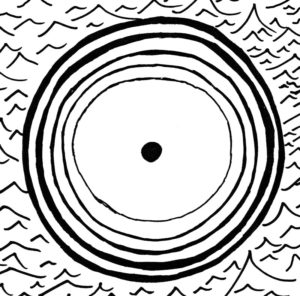
We’re trying to find the amount of time it takes for a ball to resurface after hitting water. The simplest way to do this is to make a simulation of all the forces acting on the ball. The buoyant forces, given by the second equation require you to calculate the amount of water the ball has displaced, so you must calculate the volume of a sphere cap. The volume of a sphere cap is given by the fourth equation. The third equation, for drag, requires the frontal area of the sphere cap, which is given by the fifth equation. We put these together to get a simulation. The answer is the time in between a peak and a trough. This time actually fluctuates a bit. Our answer is that the average frequency is about one ripple every 0.3969 seconds.
\vec F_G=1kg g\ down\\[8pt] \vec F_B=\frac{998.2kg gV_w}{m^3}\ up\\[8pt] \vec F_d=\frac{469.2kgA_f\vec V^2}{m^3}\ up\\[16pt] V_w=\pi rh^2-\frac{\pi h^3}3\\[8pt] A_f={\pi r^2\over(r-d)^2}-\piSolution to Fascinating Frequencies 4
We’re trying to find the frequency of Bob’s bungee jump. There is no need to make a new simulation. Simply add a few more lines of code and you will find the answer. Surprisingly, each bounce takes only approximately 0.212 seconds.
Solution to Didactic Dice
We are trying to find the average number of moves of a game. The game is big enough that we can approximate the probabilities with a bell curve. We could also use a simulation. Both give an accurate enough result. We do not need the exact answer. The average roll moves you 3.5 spaces. The probability that you land on the star on any given pass is thus 2/7, and miss it, 5/7. So the average number of passes you make is 5/(7-5) = 5/2 = 2.5 passes. 2.5*24 equals 60 squares. The average number of rolls will then be 60/3.5 equals 17 and a seventh rolls. The standard deviation for this is calculated by the formula below, and it gives about 7.071. What we do next is surprisingly simple: subtract the average number of turns it takes to move standard deviation from the average. In equation form, 17.143-7.071/3=Our answer is that the game takes an average of about 14.79 turns.
D=\sqrt{\frac{qty\times(s^2-1)}{12}}\\[8pt] D=5\sqrt2\approx7.071Solution to Fascinating Frequencies 3
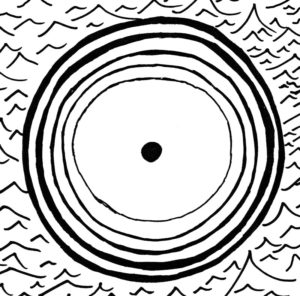
We’re trying to find the frequency of ripples on water at 20 °C from a stone. When the stone hits the water, it has a kinetic energy of 0.5 m2kg/s2. We get this from the equation KE=mv2/2. The water it passes through has a density of ≈0.9982 kg/m3. At T=0, the ripple will begin. When the stone is completely submerged, the first ripple will end. The time between is the frequency of the ripples. But how long will it take the ball to submerge? The deeper it is, the more water it must push. But the slower it goes, the less energy required to push it. There is always the force of gravity on the ball. The ball weighs 1kg and will need to displace ≈1333π cm3 of water. The volume of a sphere is 4/3πr3. This actually weighs ≈4.1802 kilograms, far more than the stone. The stone will float! The buoyant forces acting on the rock will push it upwards, with a force equal to the weight of the water displaced. The water will slow it down according to the drag equation, Cd*p*A*v2/2, where Cd is the drag coefficient, p is the density of the fluid, A is the frontal area, and v is the velocity relative to the fluid. The drag coefficient for a sphere is 0.47. The density of water is 0.9982 kg/m3. So drag is taken care of. Finally, we have the force of gravity, pushing down with the weight of the rock. Calculating the time it takes for the rock’s velocity to fall to 0 will be another challenge, Gangbuster Geometry.
Solution to Ubiquitous Units
We’re trying to solve the system of equations left in Fascinating Frequencies 2. To start, factor out v in the second equation. We do this by multiplying both sides by ms/4πd. Then we combine the 2 equations. Next, substitute in the gravitational constant G and factor out d to find the distance. We factor out d by multiplying both sides by 72000s*d3/π and taking the fourth root of both sides. Substitute d into the first equation to solve for v. Our answer is approximately 8,032 metres per second.
\vec v=\frac{2\pi d}{72\,000s}\\[8pt] \frac{4\pi\vec vd}{ms}=\frac{Gm_1}{d^2}\\[8pt] G=\frac{6.674m^3}{10^{11}s^2kg}\\[8pt] \vec v=\frac{Gmm_1s}{4\pi d^3}=\frac{\pi d}{36\,000s}\\[8pt] \vec d\approx\sqrt[4]{\frac{2.403m^4m_1s^2}{4\times10^5\pi^2s^2kg}-1}\\[8pt] \vec v\approx\sqrt[4]{\frac{\pi^2m^4m_1s^2}{11.99 \times10^8\pi^4s^6kg}-1}\\[8pt] \vec v\approx\sqrt[4]{\frac{4.162\times10^{15}m^4}{\pi^2s^4}}=8.032\times10^3\frac msSolution to Sophisticated Shapes
The challenge is to find the instantaneous acceleration of a point on a sphere when it touches the larger sphere it is rolling inside of. Before finding the acceleration of a point on the circumference of the circle, let’s find that of the centre. The radius of the larger circle is 1 metre. The centre of the small circle is not on the circumference though. It is 25cm away because the small circle has radius 25cm. This means the centre of the smaller centre is 100-75 = 25 centimetres away from that of the larger one. The smaller circle completes a rotation whose circumference is 2π*75cm = 150πcm every 4 seconds. It’s velocity is thus 375π millimetres per second sideways. This velocity changes, and it’s vector’s endpoint will naturally also complete a full circular rotation each second. This circle has radius 1.5π m/s and thus circumference 2π*375π mm/s = 75π2 centimetres per second inward. To complete this circle every second, the acceleration must be 75π2 cm/s2. The same rule applies to smaller circle. The acceleration equals 4π2r/s2 inward times the rate of rotation. For the smaller circle, this gives us 1π2 m/s2 toward it’s centre. When the point touches the rim, these vectors will align, and the point will feel their cumulative acceleration. It’s counterintuitive that the point experiences the most force when it stops, but it’s true. Add 1π2 m/s2 inward and 75π2 cm/s2 inward together to get our answer, 175π2 cm/s2 inward.